Cells’ Electric Fields Keep Nanoparticles at Bay, Scientists Confirm
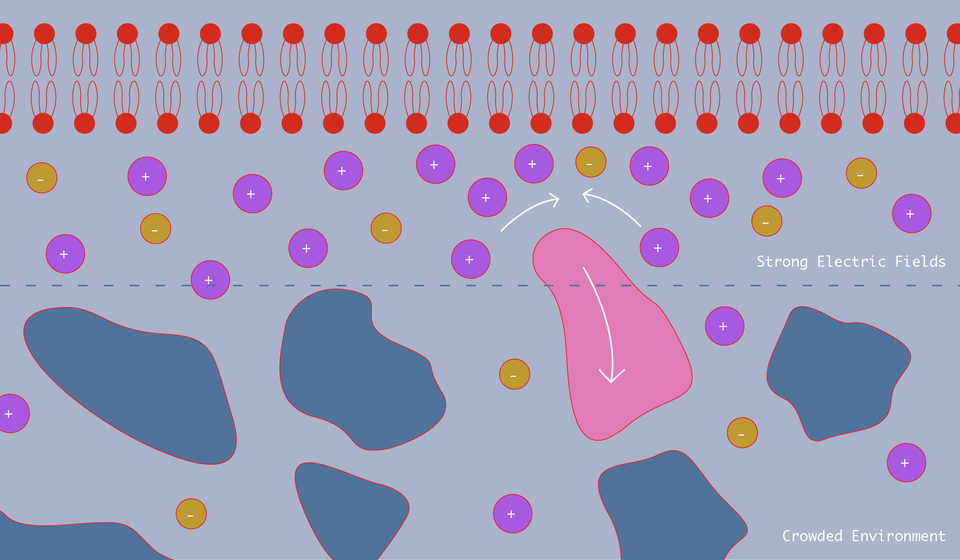
Cell membranes generate powerful electric field gradients that are largely responsible for repelling nano-sized particles like proteins from the surface of the cell — a repulsion that notably affects uncharged nanoparticles. In this schematic drawing, a negatively charged membrane (at top, in red) attracts small, positively charged molecules (purple circles), which crowd the membrane and push away a far larger, neutral nanoparticle (pink).
Credit: N. Hanacek/NIST
The humble membranes that enclose our cells have a surprising superpower: They can push away nano-sized molecules that happen to approach them. A team including scientists at the National Institute of Standards and Technology (NIST) has figured out why, by using artificial membranes that mimic the behavior of natural ones. Their discovery could make a difference in how we design the many drug treatments that target our cells.
The team’s findings, which appear in the Journal of the American Chemical Society, confirm that the powerful electrical fields that cell membranes generate are largely responsible for repelling nanoscale particles from the surface of the cell. This repulsion notably affects neutral, uncharged nanoparticles, in part because the smaller, charged molecules the electric field attracts crowd the membrane and push away the larger particles. Since many drug treatments are built around proteins and other nanoscale particles that target the membrane, the repulsion could play a role in the treatments’ effectiveness.
The findings provide the first direct evidence that the electric fields are responsible for the repulsion. According to NIST’s David Hoogerheide, the effect deserves greater attention from the scientific community.
“This repulsion, along with the related crowding that the smaller molecules exert, is likely to play a significant role in how molecules with a weak charge interact with biological membranes and other charged surfaces,” said Hoogerheide, a physicist at the NIST Center for Neutron Research (NCNR) and one of the paper’s authors. “This has implications for drug design and delivery, and for the behavior of particles in crowded environments at the nanometer scale.”
Membranes form boundaries in nearly all kinds of cells. Not only does a cell have an outer membrane that contains and protects the interior, but often there are other membranes inside, forming parts of organelles such as mitochondria and the Golgi apparatus. Understanding membranes is important to medical science, not least because proteins lodged in the cell membrane are frequent drug targets. Some membrane proteins are like gates that regulate what gets into and out of the cell.
The region near these membranes can be a busy place. Thousands of types of different molecules crowd each other and the cell membrane — and as anyone who has tried to push through a crowd knows, it can be tough going. Smaller molecules such as salts move with relative ease because they can fit into tighter spots, but larger molecules, such as proteins, are limited in their movements.
This sort of molecular crowding has become a very active scientific research topic, Hoogerheide said, because it plays a real-world role in how the cell functions. How a cell behaves depends on the delicate interplay of the ingredients in this cellular “soup.” Now, it appears that the cell membrane might have an effect too, sorting molecules near itself by size and charge.
“How does crowding affect the cell and its behavior?” he said. “How, for example, do molecules in this soup get sorted inside the cell, making some of them available for biological functions, but not others? The effect of the membrane could make a difference.”
While researchers commonly use electric fields to move and separate molecules — a technique called dielectrophoresis — scientists have paid scant attention to this effect at the nanoscale because it takes extremely powerful fields to move nanoparticles. But powerful fields are just what an electrically charged membrane generates.
“The electric field right near a membrane in a salty solution like our bodies produce can be astoundingly strong,” Hoogerheide said. “Its strength falls off rapidly with distance, creating large field gradients that we figured might repel nearby particles. So we used neutron beams to look into it.”
Neutrons can distinguish between different isotopes of hydrogen, and the team designed experiments that explored a membrane’s effect on nearby molecules of PEG, a polymer that forms chargeless nano-sized particles. Hydrogen is a major constituent of PEG, and by immersing the membrane and PEG into a solution of heavy water — which is made with deuterium in place of ordinary water’s hydrogen atoms — the team could measure how closely the PEG particles approached the membrane. They used a technique known as neutron reflectometry at the NCNR as well as instruments at Oak Ridge National Laboratory.
Together with molecular dynamics simulations, the experiments revealed the first-ever evidence that the membranes’ powerful field gradients were the culprit behind the repulsion: The PEG molecules were more strongly repelled from charged surfaces than from neutral surfaces.
While the findings do not reveal any fundamentally new physics, Hoogerheide said, they do show well-known physics in an unexpected place, and that should encourage scientists to take notice — and explore it further.
“We need to add this to our understanding of how things interact at the nanoscale,” he said. “We’ve demonstrated the strength and significance of this interaction. Now we need to investigate how it affects these crowded environments where so much biology happens.”
Paper: M. Aguilella-Arzo, D.P. Hoogerheide, M. Doucet, H. Wang and V.M. Aguilella. Charged biological membranes repel large neutral molecules by surface dielectrophoresis and counterion pressure. Journal of the American Chemical Society. Published online Jan. 16, 2024. DOI: 10.1021/jacs.3c12348
Legal Disclaimer:
EIN Presswire provides this news content "as is" without warranty of any kind. We do not accept any responsibility or liability for the accuracy, content, images, videos, licenses, completeness, legality, or reliability of the information contained in this article. If you have any complaints or copyright issues related to this article, kindly contact the author above.
